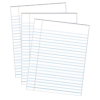
Terrain geology
Storyboard 
The geological analysis of the terrain is essential for assessing its stability and the potential to access groundwater. Public geological data sources are consulted, and field-collected data are analyzed to develop a detailed model of the soil types and their distribution. This model enables the study of soil behavior, potential movement, and the flow of water through the terrain.
ID:(160, 0)
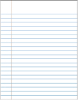
Geological studies
Description 
In Chile, important geological studies have been conducted by institutions such as the National Geology and Mining Service (SERNAGEOMIN) and the Natural Resources Information Center (CIREN). According to these studies, the Palos Verdes area and its surroundings feature the following geological units:
Details of geological units with the approximate location of the parcel (marked in red)
Geological Units
Code | Description | Geological Age | Years (My) |
PzTrbm(a) | Pelitic to semi-pelitic schists of the Bahía Mansa Metamorphic Complex | Paleozoic | 541-252 |
PzTrbm(b) | Mafic schists of the Bahía Mansa Metamorphic Complex | Paleozoic | 541-252 |
Msd1 | Sequences of deep marine sediments | Miocene | 23-16 |
Msd2 | Sequences of marine and continental sediments | Miocene | 23-5.3 |
Plfe | Fluvio-estuarine deposits | Pleistocene | 2.58-0.01 |
Reference
[1] Geology of the Valdivia-Corral Area, National Geology Subdirectorate, SERNAGEOMIN, 2012.
ID:(964, 0)
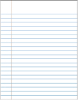
PzTrbm(a)
Description 
Description: Pelitic to semi-pelitic schists of the Bahía Mansa Metamorphic Complex
Geological Age: Paleozoic (541 to 252 million years ago)
Main Characteristics
Low to medium-grade metamorphic rocks, primarily composed of schists derived from pelitic (clay-rich) and semi-pelitic sediments. These rocks formed during the Paleozoic and are characteristic of the Los Ríos region in Chile. They are notable for their low permeability and high mechanical resistance due to compaction and recrystallization.
Porosity [2] | 2 - 5 | % | Low porosity due to compaction and recrystallization during the metamorphic process. |
Elasticity [1] | 10 - 20 | GPa | High stiffness due to the crystalline nature of the metamorphic rocks. |
Hardness [1] | 4 - 6 | Mohs | Moderate to high, influenced by minerals such as quartz and micas. |
Compressive Strength [1] | 50 - 100 | MPa | High resistance, typical of compacted metamorphic rocks. |
Permeability [2] | 1E-9 - 1E-7 | m/s | Very low, significantly limiting water flow through these rocks. |
Storage Capacity [1] | Low (<0.02) | Sy | Low, due to limited effective porosity. |
References
[1] Mahan, K. H., Goncalves, P., & Bell, T. H. (2006). Deformation and fluid flow in low-grade metamorphic rocks. Journal of Metamorphic Geology.
[2] Chester, F. M., & Logan, J. M. (1986). Implications for fluid transport in fault zones. Journal of Geophysical Research.
ID:(965, 0)
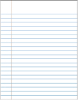
PzTrbm(b)
Description 
Description: Mafic schists of the Bahía Mansa Metamorphic Complex
Geological Age: Paleozoic (541 to 252 million years ago)
Main Characteristics
Low to medium-grade metamorphic rocks, primarily composed of schists derived from mafic rocks, rich in ferromagnesian minerals such as iron and magnesium. These rocks formed during the Paleozoic and are distributed in NW-SE trending bands in the Los Ríos region. Their composition and metamorphic grade provide high mechanical strength and low permeability.
Porosity [2] | 3 - 8 | % | Low porosity due to compaction and recrystallization during metamorphism. |
Elasticity [1] | 15 - 25 | GPa | High stiffness, characteristic of crystalline metamorphic rocks. |
Hardness [1] | 5 - 7 | Mohs | Moderate to high, due to the presence of ferromagnesian minerals such as amphiboles and pyroxenes. |
Compressive Strength [1] | 70 - 150 | MPa | High, typical of compact and metamorphic rocks. |
Permeability [2] | 1E-8-1E-6 | m/s | Very low, significantly limiting water flow through these rocks. |
Storage Capacity [1] | Low (<0.02) | Sy | Low storage capacity, due to limited effective porosity. |
References
[1] Mahan, K. H., Goncalves, P., & Bell, T. H. (2006). Deformation and fluid flow in low-grade metamorphic rocks. Journal of Metamorphic Geology.
[2] Chester, F. M., & Logan, J. M. (1986). Implications for fluid transport in fault zones. Journal of Geophysical Research.
ID:(966, 0)
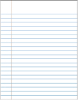
Plfe
Description 
Description: Fluvio-estuarine deposits
Geological Age: Pleistocene (2.58 million to 11,700 years ago)
Main Characteristics
Unconsolidated sediments composed of sands, silts, and clays, deposited in fluvial and estuarine environments during the Pleistocene. These deposits are typically found in low-lying and flat areas such as valleys and estuaries, serving as natural reservoirs for groundwater due to their high porosity and permeability.
Porosity [2] | 25 - 45 | % | High porosity, ideal for storing and transporting groundwater. |
Elasticity [1] | 0.1 - 1.0 | GPa | Low elasticity due to the granular, unconsolidated nature of the sediments. |
Hardness [1] | 2 - 4 | Mohs | Low hardness, composed of softer materials such as silts, clays, and sands. |
Compressive Strength [1] | 0.1 - 5 | MPa | Fragile, characteristic of unconsolidated sediments. |
Permeability [2] | 1E-3 - 1E-5 | m/s | High permeability, facilitating groundwater flow and recharge. |
Storage Capacity [1] | Medium to high (0.1 - 0.3) | Sy | High storage capacity, typical of loose and highly porous sediments. |
References
[1] Terzaghi, K., Peck, R. B., & Mesri, G. (1996). Soil Mechanics in Engineering Practice.
[2] Freeze, R. A., & Cherry, J. A. (1979). Groundwater. Prentice Hall.
ID:(967, 0)
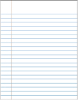
Msd1
Description 
Description: Sequences of Deep Marine Sediments
Geological Age: Miocene (23 to 16 million years ago)
Main Characteristics
Fine-grained deposits formed in deep marine environments, primarily composed of silts and clays. These sequences are characterized by low permeability and high compaction, significantly restricting water mobility within these sediments.
Porosity [2] | 5 - 15 | % | Low porosity due to compaction in deep marine environments, limiting water retention capacity. |
Elasticity [1] | 0.2 - 1.0 | GPa | Moderately low elasticity, associated with the fine nature of sediments and partial consolidation. |
Hardness [1] | 2 - 4 | Mohs | Soft, dominated by silts and clays, with minimal presence of hard minerals like quartz. |
Compressive Strength [1] | 0.5 - 3 | MPa | Low strength, typical of compacted but not fully consolidated sediments. |
Permeability [2] | 1E-5 - 1E-7 | m/s | Extremely low, significantly restricting water flow through the material. |
Storage Capacity [1] | Medium to low (<0.05) | Sy | Limited, due to low effective porosity and the compact nature of the sediments. |
References
[1] Allen, J. R. L. (1985). Principles of Physical Sedimentology. Springer.
[2] Bear, J. (1972). Dynamics of Fluids in Porous Media. Dover Publications.
[3] Blatt, H., Middleton, G., & Murray, R. (1980). Origin of Sedimentary Rocks. Prentice Hall.
ID:(971, 0)
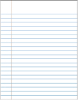
Msd2
Description 
Description: Sequences of Marine and Continental Sediments
Geological Age: Miocene (23 to 5.3 million years ago)
Main Characteristics
Strata composed of marine and continental sediments, primarily sandstones, siltstones, and conglomerates, deposited during the Miocene. These sequences reflect significant changes in sea levels and climatic conditions of the time, resulting in a mix of materials with varying degrees of compaction.
Porosity [2] | 15 - 30 | % | Intermediate porosity, typical of partially compacted sediments. |
Elasticity [1] | 0.5 - 5.0 | GPa | Low to moderate elasticity, due to granular structure and lower consolidation levels. |
Hardness [1] | 3 - 5 | Mohs | Moderate, with harder components like quartz in sandstones and softer ones like silts. |
Compressive Strength [1] | 1 - 10 | MPa | Moderate to low, characteristic of partially consolidated sediments. |
Permeability [2] | 1E-5 - 1E-7 | m/s | Moderate permeability, suitable for intermediate-depth aquifers. |
Storage Capacity [1] | Medium to high (0.05 - 0.15) | Sy | Medium to high, useful for groundwater reservoirs. |
References
[1] Allen, J. R. L. (1985). Principles of Physical Sedimentology. Springer.
[2] Bear, J. (1972). Dynamics of Fluids in Porous Media. Dover Publications.
ID:(968, 0)
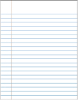
Stability parameters
Description 
Given that the Msd1 and Msd2 layers are situated above the PzTrbm(a) base, it can be assumed that these layers could present instability, sliding over the base due to the action of gravity, rainwater, or wind, which may transport the sediments to this area.
To understand the movements that may have occurred, the ranges of key parameters for Msd1, Msd2, and their mix, both in consolidated and unconsolidated states, are presented below. The information is summarized as follows:
Property | Msd1 (NC) | Msd1 (C) | Msd2 (NC) | Msd2 (C) | Mix (NC) | Mix (C) |
Cohesion (kPa) | 5-20 | 40-60 | 20-50 | 80-150 | 15-40 | 50-100 |
Internal friction angle (°) | 20-30 | 30-35 | 25-35 | 35-40 | 22-33 | 30-38 |
Unit weight (kN/m3) | 18-20 | 21-23 | 20-22 | 22-24 | 19-21 | 21-23 |
Permeability (m/s) | 1E-5-1E-7 | 1E-6-1E-8 | 1E-7-1E-8 | 1E-8-1E-10 | 1E-6-1E-8 | 1E-8-1E-9 |
Porosity (%) | 35-50 | 25-35 | 30-40 | 15-25 | 30-45 | 20-30 |
Elastic modulus (MPa) | 5-15 | 20-50 | 10-30 | 50-100 | 7-20 | 30-70 |
Compressive strength (kPa) | <1000 | 2000-5000 | <2000 | 5000-15000 | 1500-3000 | 4000-10000 |
Typical moisture (%) | 20-40 | 10-20 | 15-30 | 5-15 | 18-35 | 8-18 |
C: Consolidated, NC: unconsolidated
To estimate the behavior of the Msd1 and Msd2 layers, it is essential to study their stability. This is achieved by calculating the factor of safety $FS$, which compares the resisting forces to the sliding forces.
The resisting forces are determined by the cohesion of the material $c$, the normal stress $\sigma$ minus the pore water pressure $p$, weighted by the tangent of the internal friction angle $\phi$. On the other hand, the sliding forces are represented by the shear stress $\tau$. The relationship is expressed as:
$FS = \displaystyle\frac{c + (\sigma - p)\tan\phi}{\tau}$
Knowing the unit weight of the material $\gamma$, the unit weight of water $\gamma_w$, the thickness of the layer $z$, the degree of saturation $m$, and the slope of the hill $\theta$, the key terms can be determined as follows:
Normal stress $\sigma$:
$\sigma = \gamma z \cos\theta$
Pore water pressure $p$:
$p = m \gamma_w z$
Shear stress $\tau$:
$\tau = \gamma z \sin\theta$
The value of the factor of safety $FS$ allows classifying the stability of the layers according to the following criteria:
FS > 1.5 | Stable (under static conditions) |
1 < FS < 1.5 | Marginally stable (risk of failure) |
FS < 1 | Unstable (failure likely) |
References
[1] Principles of Physical Sedimentology, Allen, J. R. L.. Springer (1985)
[2] Details on physical and mechanical properties of marine and continental sedimentary deposits., Bear, J., Dynamics of Fluids in Porous Media. Dover Publications. (1972).
[3] Provides information on porosity, permeability, and fluid transport in porous media. Terzaghi, K., Peck, R. B., & Mesri, G., Soil Mechanics in Engineering Practice (3rd ed.). Wiley. (1996).
[4] Standard reference for mechanical properties of soils and slope stability. Lambe, T. W., & Whitman, R. V., Soil Mechanics. Wiley (1969).
[5] Main source for cohesion, internal friction, and elastic modulus properties in consolidated and unconsolidated soils.
Goudie, A. S., Encyclopedia of Geomorphology. Routledge. (2004).
[6] Explains sedimentary and geomorphological processes affecting marine deposits like Msd1 and Msd2. Bowles, J. E., Foundation Analysis and Design (5th ed.). McGraw-Hill, (1996)
[7] Reference used for mechanical properties of soils and stability analysis. Blight, G. E., Mechanics of Residual Soils. Taylor & Francis. (1997).
ID:(988, 0)
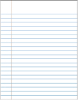
Consolidation rate
Description 
The consolidation time depends on the permeability $k$ and the compressibility index $m_v$. Using the unit weight of water $\gamma_w$, the coefficient of consolidation $c_v$ can be calculated using the formula:
$c_v = \displaystyle\frac{k}{\gamma_w m_v}$
Using Terzaghi's one-dimensional consolidation theory, a dimensionless time factor $T_v$, related to the degree of consolidation $U$, is introduced. Typical values are as follows:
Degree of Consolidation | Time Factor |
50% | 0.197 |
90% | 0.848 |
95% | 1.131 |
The consolidation time for a given degree of consolidation is calculated as:
$t = \displaystyle\frac{T_v}{c_v} H^2$
where $H$ is the thickness of the layer.
For the Msd1 and Msd2 layers, considering a 10-meter layer and 90% consolidation, the following values are obtained:
Parameter | Msd1 NC | Msd2 NC |
Permeability (m/s) | 1E-6 | 1E-8 |
Coefficient of Consolidation (m2/s) | 3.4E-3 | 1.02E-7 |
Time (90% consolidation) | 6.9 hours | 26.3 years |
ID:(990, 0)
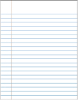
Stability of layers
Description 
The analysis of safety factors for the layers Msd1 NC, Msd1 C, Msd2 NC, Msd2 C, Mix NC, and Mix C as a function of the slope of the hill $\theta$ allows us to estimate both the probable past and future evolution of the studied hillside. This approach is key to proposing a model that explains the current situation.
By consistently plotting the curves corresponding to the extreme values of cohesion and internal friction angles, the following graphs are obtained:
Msd1 Non-Consolidated
Safety factor as a function of slope
The Msd1 non-consolidated layer becomes unstable when the slope exceeds approximately 11 degrees. In this case, as the slope ranges from 10 to over 20 degrees, this layer tends to slide and cover the underlying layer.
Msd1 Consolidated
Safety factor as a function of slope
The Msd1 consolidated layer is stable if the slope is less than 25 degrees. This indicates that the current slope has reached a stable point for this layer. Therefore, no further landslides are expected.
Msd2 Non-Consolidated
Safety factor as a function of slope
The Msd2 non-consolidated layer is stable for slopes below approximately 18 degrees, suggesting that it was originally more stable than the Msd1 layer and thus experienced fewer landslides. Additionally, being located above the Msd1 layer, its landslides would have tended to drag the Msd1 layer, forming a mixture of both.
Msd2 Consolidated
Safety factor as a function of slope
The Msd2 consolidated layer is stable for all possible slopes, which indicates that, at present, it should no longer generate landslides.
Mix Non-Consolidated
Safety factor as a function of slope
The mixture of both layers in a non-consolidated stage has a critical slope angle of 15 degrees. This means that the mixtures will settle in the lower parts of the slope where the gradient is less steep.
Mix Consolidated
Safety factor as a function of slope
The consolidated mixture is again stable, indicating that, in the final stage, it would no longer generate landslides.
Analysis Conclusions
Stability comparison:
• The Msd1 layer is clearly the most unstable, especially in its non-consolidated state, compared to Msd2 and the Mix of both layers.
• When the slope is below 20 degrees, the system tends to reach significant stability.
Inferred evolution:
• In an initial stage, the Msd1 layer experienced the most significant landslides, forming a relatively thick layer over the PzTrbm(a) base.
• Subsequently, minor landslides occurred in the Msd2 layer, leading to a second deposition stage composed primarily of Msd2 or a Mix of both layers.
• At present, with most slopes below 20 degrees, the system exhibits a predominantly stable state.
ID:(989, 0)